Cellular Senescence: Difference between revisions
No edit summary |
No edit summary |
||
Line 35: | Line 35: | ||
# '''Replicative Senescent Cells''': These cells have become senescent due to repeated cycles of replication and the consequent telomere shortening. When telomeres reach a critically short length, the cell undergoes replicative senescence. | # '''Replicative Senescent Cells''': These cells have become senescent due to repeated cycles of replication and the consequent telomere shortening. When telomeres reach a critically short length, the cell undergoes replicative senescence. | ||
# '''Oncogene-Induced Senescent (OIS) Cells''': These cells enter senescence due to the activation or overexpression of oncogenes, which are genes with the potential to cause cancer. This senescence acts as a protective mechanism, preventing potential tumorigenesis. | # '''Oncogene-Induced Senescent (OIS) Cells''': These cells enter senescence due to the activation or overexpression of oncogenes, which are genes with the potential to cause cancer. This senescence acts as a protective mechanism, preventing potential tumorigenesis. | ||
# '''DNA Damage-Induced Senescent Cells''': Exposure to agents or factors that cause DNA damage, such as radiation, certain chemicals, or oxidative stress, can induce cells to enter a senescent state as a response to protect against potential malignancies or functional aberrations. | # '''DNA Damage-Induced Senescent Cells''': Exposure to agents or factors that cause DNA damage, such as radiation, certain chemicals, or [[Oxidative Stress|oxidative stress]], can induce cells to enter a senescent state as a response to protect against potential malignancies or functional aberrations. | ||
# '''Stress-Induced Premature Senescence (SIPS)''': Various forms of cellular stress, other than DNA damage or oncogene activation, can lead to premature senescence. This includes factors like oxidative stress, mitochondrial dysfunction, or even certain drugs and therapeutic treatments. | # '''Stress-Induced Premature Senescence (SIPS)''': Various forms of cellular stress, other than DNA damage or oncogene activation, can lead to premature senescence. This includes factors like [[Oxidative Stress|oxidative stress]], [[Mitochondrial Dysfunction|mitochondrial dysfunction]], or even certain drugs and therapeutic treatments. | ||
# '''Wound Healing-Associated Senescent Cells''': These cells arise as a part of the wound healing process. They can help in tissue repair but might need to be cleared afterward to restore tissue functionality fully. | # '''Wound Healing-Associated Senescent Cells''': These cells arise as a part of the wound healing process. They can help in tissue repair but might need to be cleared afterward to restore tissue functionality fully. | ||
# '''Developmentally Programmed Senescent Cells''': These are cells that become senescent during embryonic development and play a role in shaping tissues and organs. After fulfilling their function, they are typically cleared from the body. | # '''Developmentally Programmed Senescent Cells''': These are cells that become senescent during embryonic development and play a role in shaping tissues and organs. After fulfilling their function, they are typically cleared from the body. | ||
Line 46: | Line 46: | ||
During early to mid-life, several factors contribute to the exponential accumulation of senescent cells: | During early to mid-life, several factors contribute to the exponential accumulation of senescent cells: | ||
# '''Natural Aging Process''': As individuals advance in age, cellular stresses, such as DNA damage, telomere shortening, and oxidative stress, become more prevalent, pushing more cells into the senescent state. | # '''Natural Aging Process''': As individuals advance in age, cellular stresses, such as DNA damage, [[Telomere Attrition|telomere shortening]], and [[Oxidative Stress|oxidative stress]], become more prevalent, pushing more cells into the senescent state. | ||
# '''Compounding Effects''': As more cells become senescent, the senescence-associated secretory phenotype (SASP) can induce senescence in neighboring cells, creating a compounding effect where the rate of new cells entering senescence increases over time. | # '''Compounding Effects''': As more cells become senescent, the senescence-associated secretory phenotype (SASP) can induce senescence in neighboring cells, creating a compounding effect where the rate of new cells entering senescence increases over time. | ||
# '''Environmental and Lifestyle Factors''': Repeated exposure to stressors like ultraviolet radiation, toxins, or an unhealthy lifestyle can further accelerate the accumulation of senescent cells during these years. | # '''Environmental and Lifestyle Factors''': Repeated exposure to stressors like ultraviolet radiation, toxins, or an unhealthy lifestyle can further accelerate the accumulation of senescent cells during these years. | ||
Line 52: | Line 52: | ||
However, it's not well-established that the growth rate of senescent cells declines substantially in advanced age. The reasons for a potential stabilization or slowdown include: | However, it's not well-established that the growth rate of senescent cells declines substantially in advanced age. The reasons for a potential stabilization or slowdown include: | ||
# '''Depleted Stem Cell Pools''': With age, the body's pool of stem cells, responsible for tissue regeneration and repair, diminishes. Since there are fewer actively dividing cells in very elderly individuals, there may be fewer cells to enter a senescent state. | # '''[[Stem Cell Exhaustion|Depleted Stem Cell Pools]]''': With age, the body's pool of stem cells, responsible for tissue regeneration and repair, diminishes. Since there are fewer actively dividing cells in very elderly individuals, there may be fewer cells to enter a senescent state. | ||
# '''Natural Cellular Attrition''': Over time, some senescent cells might undergo natural cell death, even if they are initially resistant to apoptosis. | # '''Natural Cellular Attrition''': Over time, some senescent cells might undergo natural cell death, even if they are initially resistant to apoptosis. | ||
# '''Tissue Atrophy and Reduced Cellularity''': Some tissues lose cell density with advanced age, potentially contributing to the reduced absolute numbers of senescent cells. | # '''Tissue Atrophy and Reduced Cellularity''': Some tissues lose cell density with advanced age, potentially contributing to the reduced absolute numbers of senescent cells. |
Latest revision as of 03:12, 21 January 2024
Cellular senescence, a state in which cells lose their ability to divide and function properly, is a pivotal concept in the study of aging and longevity. This phenomenon is intricately linked with the Hayflick limit, named after biologist Leonard Hayflick, who discovered in the 1960s that most somatic cells have a limited capacity to divide, typically around 40 to 60 times, before they enter senescence. The limitation arises primarily due to telomere shortening—the protective ends of chromosomes that diminish with each cellular division. Once telomeres reach a critical length, the cell perceives it as DNA damage, prompting cell cycle arrest and thereby preventing potential genetic instability.
Cellular senescence serves as a double-edged sword. On one hand, it acts as a protective mechanism against cancer, ensuring that damaged cells don't proliferate uncontrollably. On the other, the accumulation of senescent cells contributes to aging and various age-related diseases. As the field of longevity research advances, understanding and addressing the nuances of cellular senescence will be key. Strategies that target senescence, either by removing these cells or modulating their effects, offer promising avenues for enhancing healthspan and potentially extending lifespan.
Definition and Characteristics
Cellular senescence is a cellular state characterized by a set of distinctive features that mark the end of a cell's ability to divide and function optimally. At the heart of this process is:
- Permanent Cell Cycle Arrest: In this halted state, cells lose their ability to undergo mitotic division. This is often a result of critical telomere shortening, DNA damage, or other stressors that signal the cell it should no longer replicate to prevent potential errors or malfunctions in future cell generations.
- Changes in Cell Morphology and Function: Senescent cells often undergo noticeable changes in shape, size, and internal structures. Additionally, their functionality diminishes, which can impact tissue integrity and the cellular microenvironment, leading to suboptimal organ and system performance over time.
- Senescence-Associated Secretory Phenotype (SASP): One of the defining features of senescent cells is their altered secretory profile. They release a mix of pro-inflammatory cytokines, chemokines, growth factors, and proteases. While the SASP can have beneficial roles, such as in wound healing and tissue regeneration, its chronic presence is associated with a pro-inflammatory environment, which is implicated in various age-related pathologies.
Causes
Several factors can induce cellular senescence, underscoring the multifactorial nature of this biological phenomenon:
- Telomere Shortening: One of the primary triggers for cellular senescence is the process of telomere attrition. Telomeres act as protective caps at the ends of chromosomes, ensuring DNA stability and integrity during cell division. However, with each division, these telomeres progressively shorten. Once they are eroded to a critical length, the cell recognizes it as a potential risk for DNA misrepair and genetic instability, leading it to enter a state of permanent growth arrest or senescence.
- DNA Damage: Cells are constantly exposed to various internal and external factors that can induce DNA damage. Agents like ultraviolet radiation, environmental toxins, and metabolic by-products like reactive oxygen species can cause mutations or other DNA lesions. When the damage is too extensive or irreparable, the cell, instead of proliferating with potentially faulty DNA, enters a state of senescence to prevent the propagation of these errors.
- Oncogene Activation: Oncogenes are genes that, when activated or overexpressed, can drive cells into uncontrolled growth and potentially lead to tumor formation. In certain scenarios, the activation or aberrant expression of these genes can be recognized by the cell as a precancerous signal. To counteract the risk of malignancy, the cell initiates a senescent program, effectively halting its own proliferation and thus reducing the risk of tumor development.
Additionally, other lesser-known factors like epigenetic changes, mitochondrial dysfunction, and chronic inflammation have also been implicated in driving cells toward senescence. As research advances, our understanding of these causative factors and their interplay will pave the way for more effective therapeutic interventions targeting cellular senescence.
Clearance
Senescent cells are cleared from the body primarily through the immune system, specifically by specialized immune cells that recognize and eliminate these non-dividing, functionally altered cells. Here's a detailed look at the mechanisms:
- Macrophages: One of the primary cells responsible for clearing senescent cells are macrophages. These immune cells "eat" or phagocytose senescent cells, breaking them down and eliminating them from the tissue.
- Natural Killer (NK) Cells: NK cells are another type of immune cell that can recognize and kill senescent cells. They often target cells that exhibit stress signals on their surface without displaying the normal "self" markers.
- T Cells: Some types of T cells, especially CD4+ helper T cells and CD8+ cytotoxic T cells, can recognize and help eliminate senescent cells.
- Senescence-Associated Secretory Phenotype (SASP): While the SASP is known for its pro-inflammatory properties, certain components of the SASP can attract immune cells to the location of the senescent cells, aiding in their clearance.
- Apoptosis: In some circumstances, senescent cells can undergo programmed cell death, or apoptosis. While this isn't the immune system clearing them, it's a way for the body to rid itself of these cells.
In healthy young organisms, the immune system efficiently clears senescent cells, ensuring they don't accumulate in tissues. However, as organisms age and the immune system's function declines (a phenomenon known as immunosenescence), the removal of senescent cells becomes less efficient. This reduced clearance, combined with an increased rate of cells entering senescence, can result in the accumulation of senescent cells in tissues, contributing to aging and age-related diseases.
Types and Classifications
In humans, cellular senescence is a complex process and can be induced by various stimuli or stresses. While the term "senescent cells" often refers to cells that have undergone the senescence program in response to these stresses, it's worth noting that there isn't just one type of senescent cell. Instead, there are multiple subtypes or classifications based on the cause of senescence. Here are some of the main types of senescent cells based on the inducers of senescence:
- Replicative Senescent Cells: These cells have become senescent due to repeated cycles of replication and the consequent telomere shortening. When telomeres reach a critically short length, the cell undergoes replicative senescence.
- Oncogene-Induced Senescent (OIS) Cells: These cells enter senescence due to the activation or overexpression of oncogenes, which are genes with the potential to cause cancer. This senescence acts as a protective mechanism, preventing potential tumorigenesis.
- DNA Damage-Induced Senescent Cells: Exposure to agents or factors that cause DNA damage, such as radiation, certain chemicals, or oxidative stress, can induce cells to enter a senescent state as a response to protect against potential malignancies or functional aberrations.
- Stress-Induced Premature Senescence (SIPS): Various forms of cellular stress, other than DNA damage or oncogene activation, can lead to premature senescence. This includes factors like oxidative stress, mitochondrial dysfunction, or even certain drugs and therapeutic treatments.
- Wound Healing-Associated Senescent Cells: These cells arise as a part of the wound healing process. They can help in tissue repair but might need to be cleared afterward to restore tissue functionality fully.
- Developmentally Programmed Senescent Cells: These are cells that become senescent during embryonic development and play a role in shaping tissues and organs. After fulfilling their function, they are typically cleared from the body.
The senescent state is typically characterized by a halt in cell division, resistance to apoptosis (cell death), and often the secretion of a mix of bioactive molecules known as the senescence-associated secretory phenotype (SASP). The SASP can have both beneficial and detrimental effects, depending on the context.
Accumulation with Age
Senescent cells exhibit a unique pattern of accumulation in the human body, marked by an exponential rise during mid-life followed by a reduced growth rate in advanced age. Understanding this pattern offers insights into aging processes and potential interventions.
During early to mid-life, several factors contribute to the exponential accumulation of senescent cells:
- Natural Aging Process: As individuals advance in age, cellular stresses, such as DNA damage, telomere shortening, and oxidative stress, become more prevalent, pushing more cells into the senescent state.
- Compounding Effects: As more cells become senescent, the senescence-associated secretory phenotype (SASP) can induce senescence in neighboring cells, creating a compounding effect where the rate of new cells entering senescence increases over time.
- Environmental and Lifestyle Factors: Repeated exposure to stressors like ultraviolet radiation, toxins, or an unhealthy lifestyle can further accelerate the accumulation of senescent cells during these years.
However, it's not well-established that the growth rate of senescent cells declines substantially in advanced age. The reasons for a potential stabilization or slowdown include:
- Depleted Stem Cell Pools: With age, the body's pool of stem cells, responsible for tissue regeneration and repair, diminishes. Since there are fewer actively dividing cells in very elderly individuals, there may be fewer cells to enter a senescent state.
- Natural Cellular Attrition: Over time, some senescent cells might undergo natural cell death, even if they are initially resistant to apoptosis.
- Tissue Atrophy and Reduced Cellularity: Some tissues lose cell density with advanced age, potentially contributing to the reduced absolute numbers of senescent cells.
It's essential to understand that the presence and accumulation of senescent cells in tissues are associated with age-related diseases and dysfunctions. Even if the absolute number stabilizes or slows in its increase, the detrimental effects of these cells, especially through SASP, can have significant impacts on tissue function and overall health.
Implications for Age-Related Diseases
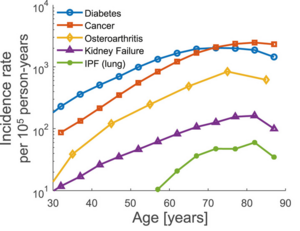
Age-related diseases are the main causes of death and disability. These diseases include cardiovascular disease, cancer, Alzheimer's disease, diabetes, kidney failure, and osteoarthritis. They affect different organ systems and have different origins, including mutations, dysregulated homeostasis, fibrosis, and degenerative processes.[1]
Despite the differences between these pathologies, they have certain universal features in terms of their incidence rate. The incidence rate of a disease is defined as the number of new cases per year divided by the size of the population. The incidence rate of each age-related disease rises roughly exponentially with age. For many of the diseases, the incidence rate then drops at very old ages. Interestingly, the slope of the rising part of the incidence curve is similar for many age-related diseases, in the range of 6–8% per year (see Figure) . This similarity hints at a common biological process of aging that governs the onset of these different diseases. It is thus of interest to develop theories for the origin of the incidence of age-related diseases, in order to detect such a common process.[1]
Implications for Aging and Disease
- Tissue Dysfunction: Senescent cells can impair tissue function due to their loss of proliferative capacity and the secretion of SASP factors, which can cause inflammation and damage surrounding cells.
- Chronic Diseases: Increased senescent cell burden is associated with various age-related diseases, including osteoarthritis, atherosclerosis, and certain types of cancers.
- Reduced Regenerative Capacity: Senescence in stem cells can reduce the body's ability to repair damaged tissues, leading to slower recovery and reduced tissue functionality.
Therapeutic Approaches Targeting Senescence
- Senolytics: These are drugs designed to selectively remove senescent cells from the body, thus reducing their negative impact. Examples include dasatinib and quercetin.
- Senomorphics: These compounds aim to modulate the SASP, reducing the harmful effects of senescent cells without necessarily removing them.
- Lifestyle Interventions: Factors like diet, exercise, and stress reduction can potentially influence the onset and accumulation of senescent cells.
Research and Future Directions
With the understanding of senescence's role in aging, there's a growing interest in developing strategies to modulate this process. Future research aims to:
- Understand the exact mechanisms driving senescence.
- Develop more targeted therapies for senescent cell removal or modulation.
- Explore the long-term effects and potential risks of senescence-targeting interventions.
See also
- Senolytics
- Wikipedia - Cellular senescence
Todo
- 2021, Senescent cells and the incidence of age-related diseases [1]
- 2019, Senescent cell turnover slows with age providing an explanation for the Gompertz law [2]
- 2019, Hallmarks of senescence and aging [3] - Senescent cell biomarkers
References
- ↑ Jump up to: 1.0 1.1 1.2 1.3 Katzir I et al.: Senescent cells and the incidence of age-related diseases. Aging Cell 2021. (PMID 33559235) [PubMed] [DOI] [Full text] Age-related diseases such as cancer, cardiovascular disease, kidney failure, and osteoarthritis have universal features: Their incidence rises exponentially with age with a slope of 6-8% per year and decreases at very old ages. There is no conceptual model which explains these features in so many diverse diseases in terms of a single shared biological factor. Here, we develop such a model, and test it using a nationwide medical record dataset on the incidence of nearly 1000 diseases over 50 million life-years, which we provide as a resource. The model explains incidence using the accumulation of senescent cells, damaged cells that cause inflammation and reduce regeneration, whose level rise stochastically with age. The exponential rise and late drop in incidence are captured by two parameters for each disease: the susceptible fraction of the population and the threshold concentration of senescent cells that causes disease onset. We propose a physiological mechanism for the threshold concentration for several disease classes, including an etiology for diseases of unknown origin such as idiopathic pulmonary fibrosis and osteoarthritis. The model can be used to design optimal treatments that remove senescent cells, suggeting that treatment starting at old age can sharply reduce the incidence of all age-related diseases, and thus increase the healthspan.
- ↑ Karin O et al.: Senescent cell turnover slows with age providing an explanation for the Gompertz law. Nat Commun 2019. (PMID 31792199) [PubMed] [DOI] [Full text] A causal factor in mammalian aging is the accumulation of senescent cells (SnCs). SnCs cause chronic inflammation, and removing SnCs decelerates aging in mice. Despite their importance, turnover rates of SnCs are unknown, and their connection to aging dynamics is unclear. Here we use longitudinal SnC measurements and induction experiments to show that SnCs turn over rapidly in young mice, with a half-life of days, but slow their own removal rate to a half-life of weeks in old mice. This leads to a critical-slowing-down that generates persistent SnC fluctuations. We further demonstrate that a mathematical model, in which death occurs when fluctuating SnCs cross a threshold, quantitatively recapitulates the Gompertz law of mortality in mice and humans. The model can go beyond SnCs to explain the effects of lifespan-modulating interventions in Drosophila and C. elegans, including scaling of survival-curves and rapid effects of dietary shifts on mortality.
- ↑ Dodig S et al.: Hallmarks of senescence and aging. Biochem Med (Zagreb) 2019. (PMID 31379458) [PubMed] [DOI] [Full text] The complex process of biological aging, as an intrinsic feature of living beings, is the result of genetic and, to a greater extent, environmental factors and time. For many of the changes taking place in the body during aging, three factors are important: inflammation, immune aging and senescence (cellular aging, biological aging). Senescence is an irreversible form of long-term cell-cycle arrest, caused by excessive intracellular or extracellular stress or damage. The purpose of this cell-cycles arrest is to limit the proliferation of damaged cells, to eliminate accumulated harmful factors and to disable potential malignant cell transformation. As the biological age does not have to be in accordance with the chronological age, it is important to find specific hallmarks and biomarkers that could objectively determine the rate of age of a person. These biomarkers might be a valuable measure of physiological, i.e. biological age. Biomarkers should meet several criteria. For example, they have to predict the rate of aging, monitor a basic process that underlies the aging process, be able to be tested repeatedly without harming the person. In addition, biomarkers have to be indicators of biological processes, pathogenic processes or pharmacological responses to therapeutic intervention. It is considered that the telomere length is the weak biomarker (with poor predictive accuracy), and there is currently no reliable biomarker that meets all the necessary criteria.